The Permeability of Soil Explained
by Dr. Kasia Zamara, on July 09, 2024
What is the permeability of soil?
The presence of water affects the properties of the soil it encounters. The permeability of a soil is the capacity of the soil to allow water to pass through it. Soil permeability is usually represented by the coefficient of permeability (k), where k is the rate of flow of water per unit area of soil when under a unit hydraulic gradient. This is an important measure for any engineering structure located on, in, or under the soil since this will impact the soil strength and stresses. For soils, we are usually concerned with water flow. The rate of flow per unit area is referred to as permeability, or sometimes as hydraulic conductivity.Leakage rates through earth dams or storage ponds clearly relate to soil permeability, but the settlement and stability of embankments are also highly dependent upon foundation soil permeability. The effectiveness of side drains along a haul road will also depend upon the permeability of the subgrade soil.
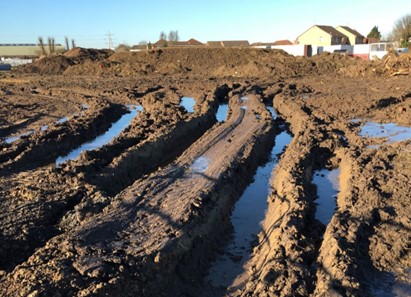.jpg)
Darcy’s Law in Relation to the Permeability of Soil
The velocity of a fluid's flow through a porous medium is directly related to the hydraulic gradient causing the flow. This is known as Darcy’s Law. Put simply, water will only flow through a soil from A to B if there is a pressure difference between A and B. The velocity of flow is directly related to the magnitude of that pressure difference. The temperature and viscosity of the fluid have an effect, as does the particle size, void content, and composition of the medium.What factors affect soil permeability?
Soils are made up of particles with interconnecting voids in between. These voids can be filled with air or water. If all voids are filled with water, the soil is said to be saturated. If only a proportion of the voids are water-filled, the soil is said to be partially saturated. Water does not pass through soil particles; it flows through the voids between particles. Water can pass through these interconnecting voids when a pressure difference exists due to a difference in water level (hydraulic head), or from the suction of plant roots as an effect of transpiration. The shape and texture of the particles, the relative volume of voids in the soil (voids ratio) and the degree of saturation all influence permeability. Compaction will clearly have an influence, as it reduces the voids ratio of the soil.Stratification of the soil also influences permeability. Where soils are built up in layers, during construction or by deposition, it is likely that permeability will be greater horizontally than vertically across the layer boundaries.
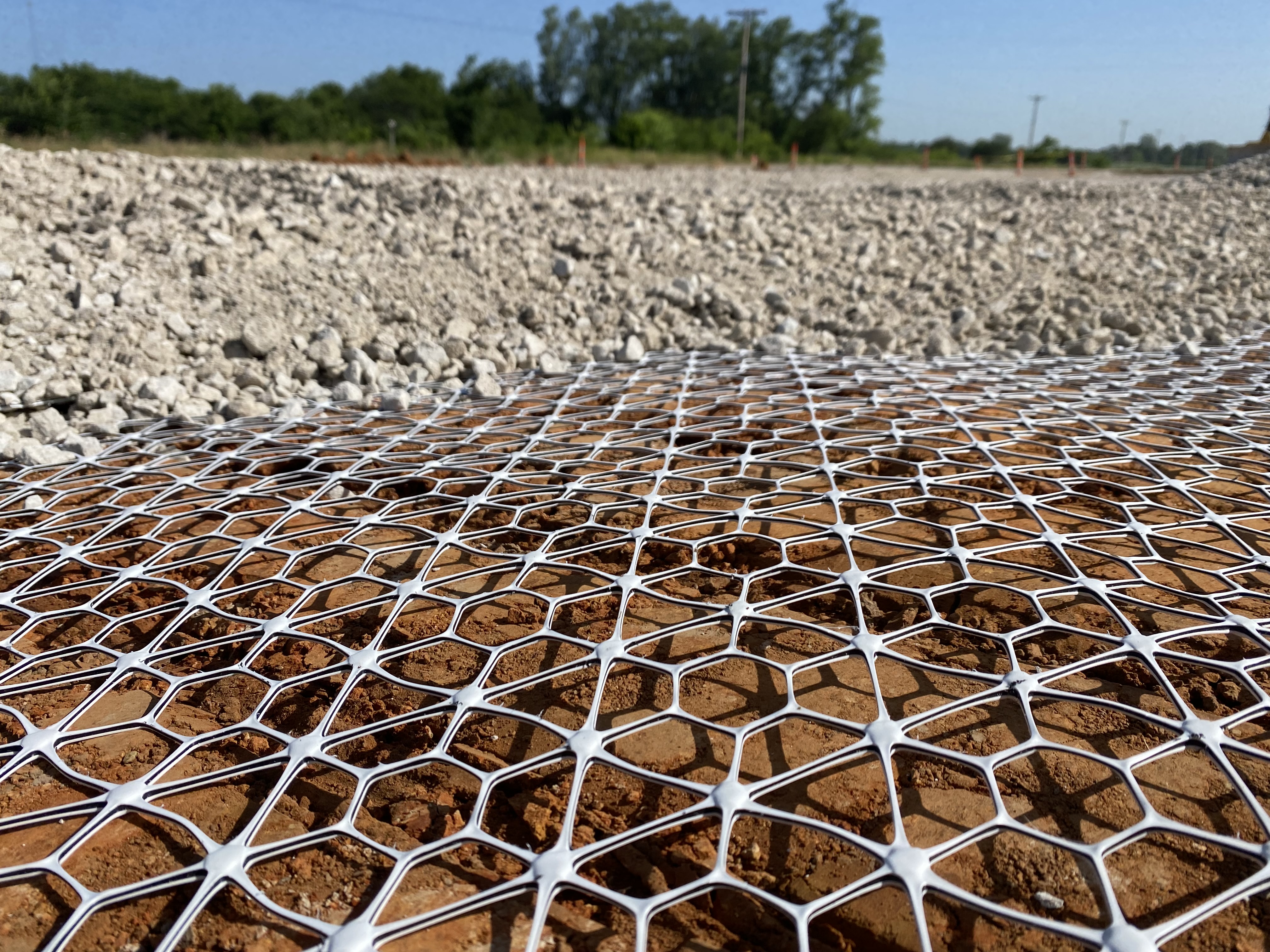
Saturated low permeability soils present problems for road construction. Using a stabilization geogrid such as Tensar InterAx geogrid, protects the weak subgrade and enables placement and compaction of the subbase above.
What is the importance of permeability of soil in civil engineering?
For earth structures, such as dams or retention ponds, the rate of leakage is clearly important, but permeability is also of critical importance for the stability of embankments. As soils are loaded, water within the soil is subject to an increase in pressure, referred to as pore pressure. Increased pore pressure reduces particle to particle contact and reduces soil strength. The excess pore pressure slowly dissipates as water flows out of the soil and the soil consolidates. The rate of pore pressure dissipation will be influenced by the permeability of the soil. Low permeability soils will take longer to consolidate. This has an impact on the rate of strength gain and also the rate of settlement, both of which are important for all earth structures. For roadways, the permeability of the subgrade soil will have a marked effect on the subgrade strength during and after construction. A saturated soil strength may therefore be more appropriate for road design, particularly during the construction phase.Soil Permeability Tests
Soil permeability testing is typically done through sample analysis. There are two types of laboratory tests: Falling Head Test and Constant Head Test. Standard test protocols are available, such as ISO. 17892-11:2019.Falling Head Test
In this soil permeability test, the differential pressure (the head) through the sample is allowed to decrease as water infiltrates the sample. Thus, there is diminishing pressure over the course of the test. This type of test is generally limited to fine-grained soils
Constant Head Test
In this test the differential pressure (the head) across the sample remains constant throughout the test. This permeability test is equally valid for gravel, sands, and clay soils.
Field Testing
Field testing can also be carried out. These are usually simple percolation tests where a hole is bored and filled with water. The time for the water level to drop is then noted as an indicator of permeability. More reliable field test methods are available, such as the pump test where water is pumped from a borehole while hydraulic head measurements are made from a series of adjacent holes to determine the subterranean hydraulic surface profile during pumping, and from that calculate a value of permeability.
Field tests are more likely to provide a more accurate indication of the permeability than laboratory tests, which may overestimate the value (indicating much lower permeabilities than the field tests). The main reason for this is that field tests would work on a large sample where ‘macro permeability’ through layers where natural fissures and soil cracks may occur. Laboratory size samples will not be large enough to become a representative of the actual layer variability. If soil permeability is a driving parameter in your design but your sources of information might be questionable, it is advisable to run sensitivity checks to verify how changes in soil permeability will affect your design.
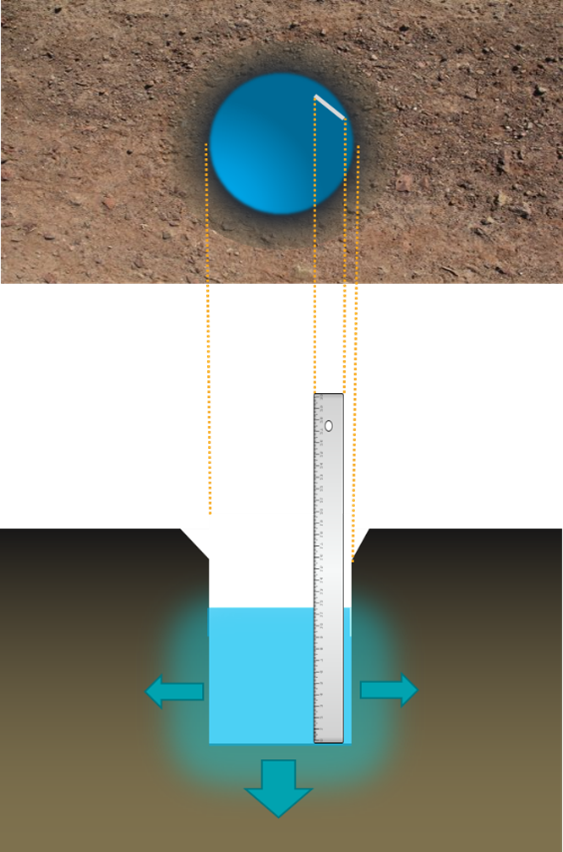
Simple Field Percolation Test – measuring the rate of drop in water level in an accurately sized hole enables calculation of saturated soil conductivity, an indicator of soil permeability.
A Marriage of Geosynthetic Materials
There have been many innovations in geosynthetic technology since the first use of geotextiles in the 1960s, both in the geosynthetic materials themselves and our understanding of their proper application. As our understanding of the functions of different geosynthetic materials has developed, it has led to the creation of geocomposite materials that combine these functions.Geogrids and geotextiles each have important functions. One of the most important geosynthetic functions, which can be accomplished using both geogrids and geotextiles, is the separation of fine-grained subgrade soils, like silts and clays, from granular layers. In the case of geogrids, separation depends on the relative size and gradation of both the granular material and the fine-grained soil. With proper design, a well-graded aggregate placed over a stiff, stable geogrid will function as a filter, preventing migration of fine-grained soils. This will provide the best solution in most cases for long-term separation of fine-grained soils from granular material.
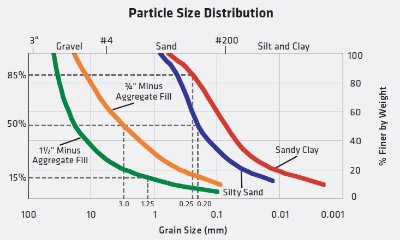
However, in some cases, filtration cannot be achieved, due to the combination of the gradation of the subgrade soils and the available granular material. In other cases, the designer does not have sufficient data on the subgrade soils to determine if filtration can be achieved. When faced with these issues, the best solution may be the use of a geotextile to ensure that separation is maintained. Geotextiles provide separation based on the openings in the fabric, which allow water to pass through the material while being small enough to restrict the passage of fine-grained soil particles. In most cases, the fabrics used for separation are light (4 to 8 ounces/square yard) non-woven geotextiles.
Because fine-grained subgrades requiring separation are almost always unstable as well, the stabilization function of geogrids is also needed. So in cases where a geotextile is specified to ensure separation, it is often installed under a geogrid, which stabilizes the granular layer above to provide a stable surface. When using this approach with separate geogrid and geotextile materials, this results in one or more added construction steps.
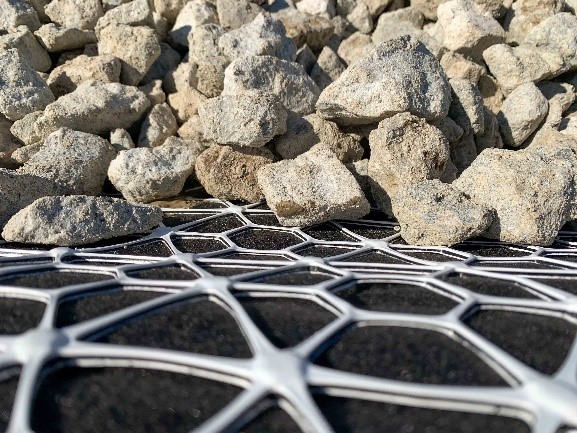
Geosynthetic technologies offer some of the greatest potential benefits for infrastructure improvement. By developing InterAx FilterGrid, a composite material that combines multiple geosynthetic functions in a single step, Tensar has added another tool for engineers and contractors to build more efficiently.